Engineering on a small scale
Engineering that takes place on small scales could have huge implications for human health.
By University Communications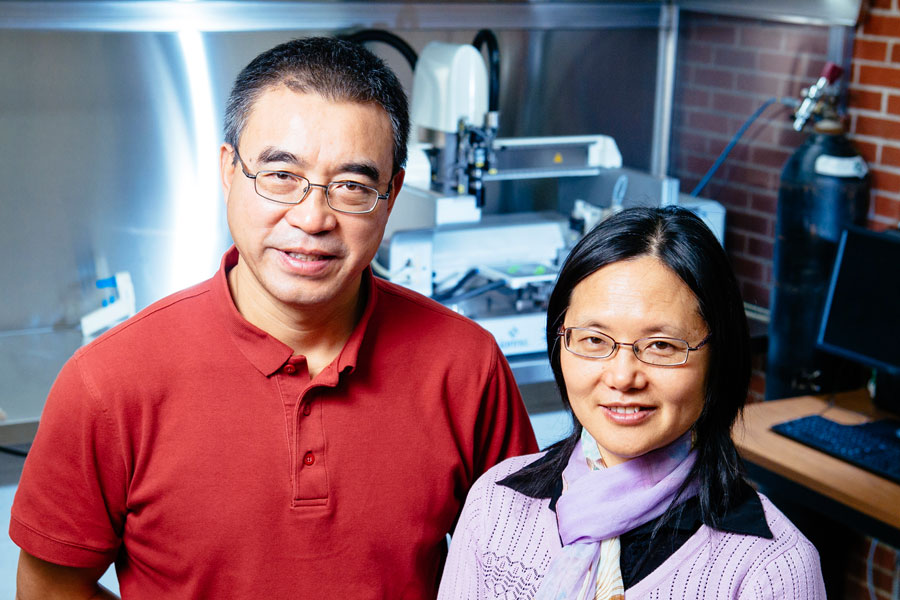
Could biomaterial scaffolds heal spinal cord injury?
Chen established the Tissue Engineering Research Group (TERG) in 2007. It is a multidisciplinary group that brings researchers from engineering and life sciences together in a quest to produce biomaterial "scaffolds" to help heal damaged tissues or organs such as spinal cord injury.
"A scaffold is a three-dimensional structure with interconnected pore networks that supports cell growth in damaged areas," Chen said. "We use the word scaffold, which is an engineering term, for a structure that helps patients build new tissues. Our research is looking for ways to build scaffolds from biodegradable, biocompatible materials that are also capable of incorporating living cells."
The principle behind Chen's research is that cells obtained from a patient's tissue could be seeded onto a scaffold, growing into functional tissues or organs that could then be implanted at the site of an injury for healing.
The implications for human health are profound. Biomaterial scaffolds could one day help the body self repair various kinds of damage.
"There are a lot of applications, but we are currently working on scaffolds for repair of peripheral nerve, articular joint cartilage (the tissue covering the ends of joint bones), spinal cord injury and damage caused by heart attack," explained Chen. "We need to fabricate different scaffolds for different tissues and organs, so we are researching what kinds of materials are best for specific applications."
Chen and his team fabricate the scaffolds in his Bio-Fabrication Lab using an advanced 3D Bioplotter.
"We start with a biomaterial solution and build the 3D scaffold layer by layer. From a technical point of view, one of the most significant challenges is fabricating a scaffold with the vascular network necessary to transport nutrients and metabolic waste. This is very important."
Another challenge is integrating the living cells into the bio-fabrication process so they retain their cellular properties and function. He relies on research collaborators in medicine and life sciences for the cells. Research to date is promising, with the scaffolds working well in models of peripheral nerve, spinal cord and heart attack damage.
Could nanocoatings lead to lifetime artificial joint replacements?
Using nanostructured coatings to improve the durability and performance of biomedical implants is just one area of Yang's research program, but it is generating great interest in the health community.
Every year in Canada, more than 60,000 people undergo hip-or knee-replacement surgery but here's the rub: the average lifespan of an artificial joint is 15-20 years.
Component wear leads to device loosening, which limits joint lifespan, said Yang. "Artificial hip and knee joints provide stability and carry body weight so they have to be strong and flexible. The biggest problems with metals are low wear resistance, high friction coefficient and limited corrosion resistance. These limit the life of artificial joints."
But what if you could apply a nanocoating that would increase wear and corrosion resistance, while keeping friction low? The possibility intrigued Yang.
"When you know the surface of materials, you can develop nanocoatings that improve wear resistance and extend material lifespans."
One thing to know about Yang's research is its scale—in her world, things are measured in nanometers (nm); one nanometer is a billionth of a meter. To put that into perspective, a human hair is about 60,000 to 80,000 nm
wide. When she applies a nanostructured coating to the surface of a material, each layer of coating is a few nanometres thick.
"The coating we have developed is a diamond-like coating combined with nanoparticles," Yang explained. "It's very low friction with really high wear resistance and corrosion resistance. It could improve the lifetime of artificial joints up to 40 years, maybe longer."
The major challenge right now is getting the nanomaterial to adhere well to the metal so it will last. But challenge is what drives most research. "For me, the most exciting part is when we overcome a challenge," Yang said, "and we overcome them one by one."
Both Chen and Yang rely on the Canadian Light Source (CLS) synchrotron to advance their research. Chen and his group have developed new synchrotron biomedical imaging technologies to characterize scaffolds and tissue samples. Yang and her team use the CLS to investigate nanostructured diamond-like films. "The nanostructure plays a key role—that is a major hurdle—and we need the CLS to know we've got the structure," said Yang.
It is hard to predict how soon tissue scaffolds and nanocoatings could become viable treatments; medical research is painstakingly slow. A process has to work consistently and predictably at every step, and researchers have to fully understand how and why it works before they can move on to the next step. Still, both Chen and Yang are hopeful they'll see their work evolve into viable treatments for improving human health from the inside out.
Bev Fast is a Saskatoon freelance writer.