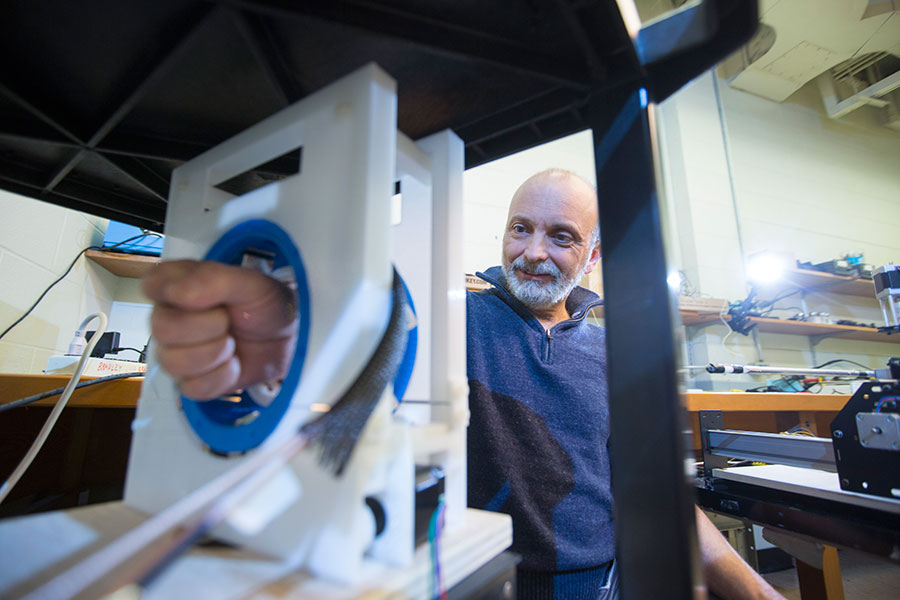
Sky-high mathematician
In six Star Trek movies and the television series of the same name, the USS Enterprise’s doctor, Leonard “Bones” McCoy, whips out his hand-held tricorder to scan the bodies of injured and ill crew members.
By dee Hobsbawn-Smith
To Gordon Sarty, Bones' tricorder represents a mind meld of his own long-held love of astronomy and the sky, and his work as a mechanical engineer with a PhD in applied mathematics.
The man who once built telescopes as a youth and dreamed of working in aerospace is on the verge of sending a portable MRI to the International Space Station, bringing high-tech medical imaging in outer space one step closer to reality. "Our ultimate objective is to make magnetic resonance imaging (MRI) portable," said Sarty (Msc'90, PhD'95), professor and acting chair of the Division of Biomedical Engineering with the College of Arts and Science.
Sarty is still building things. A typical full-size MRI machine is a large, tubular magnet, about a metre-and-a-half long and 60 centimetres across—big enough to contain a human body. In the basement of the U of S Physics Building, Sarty and two graduate students are developing and fine-tuning a smaller version, large enough to scan a human wrist, with an internal diameter of 18 centimetres and 36 centimetres long. It weighs in at a relatively slender 50 kilograms, light enough to be considered for inclusion on the International Space Station, where volume, weight and electrical needs are strictly governed. The MRI Sarty is working on is small enough to be considered portable, and at $250,000, it costs a fraction of its larger cousin's $2 million price tag.
The project was one of five that won a 2014 Canadian Space Agency competition for new medical-study instruments. Two were selected for further funding in February, but the MRI project didn't make the cut. "It boils down to risk and dollars," he said wryly. "It would be awesome that Canada would be the first ever to put an MRI in space. Canada doesn't have a whole lot of firsts in space."
Sarty remains hopeful that he will receive additional funds toward production of the MRI in next year's allocation of the space agency's budget. It becomes a tight timeline, however. The space station's international collaboration among the European Space Agency, NASA, Japan and Russia is set to expire by 2025 at the latest, and Canada has not signed on to the agreement beyond 2020. Further, by 2030, the space station itself will be allowed to burn up in the Earth's atmosphere. "It will take a couple years to build the MRI," he said. "If we start building now, by 2017, it will get three years in operation."
Sarty is frequently asked, "Why build it, and what is its use?"
"The technological angle is that it's a challenge to get an MRI light enough to put on a rocket," he said in response. "That attracted me to the problem." A full-size MRI weighs about 11 tons. He explained that it is necessary to create a "very perfect" magnetic field, that requires many neodymium magnets. In the lab, he and his team are developing new technology to enable the MRI to work with imperfect magnets. "It will make the MRI lighter, which leads to greater portability.
"The other reason is that in space, astronauts lose muscle and bone mass because they don't use them," he added. "All the studies to understand why that happens are based on before and after measurement—MRIs before and after, but none while actually flying. It has been a guess to what happens in-between." Participating astronauts on the space station would be tested monthly by MRI if and when the machine reaches the stars.
Beyond space, a smaller, less expensive and portable MRI would be a worthwhile project on planet Earth. "What's really important is to increase accessibility of MRI and eliminate long waiting lines," Sarty said. The machine would not only make diagnostic imaging more affordable, it could be put to use in remote rural and northern communities, in developing countries, war zones and in disaster relief areas.
Sarty has retained his fondness for the sky and is a long-time active member of the Royal Astronomical Society of Canada. When he's not looking at stars, working in the lab or spending time with his family, he and Associate Professor Michael Bradley are busy co-supervising three fourth-year engineering students, Mitchell Tallon, Michael Dorma and Graham Scott, as they prepare their capstone project on measuring magnetic fields.
"My focus is along a businesslike direction," Sarty said. "Within two or three years, I hope to see this [portable MRI] on the market. This is the big project I'd like to see happen, either by working with manufacturers or by solving the technological problems ourselves.
How an MRI works
Magnetic resonance imaging (MRI) is a medical technique based on radio waves, magnets and computers, used to investigate the anatomy and physiology of the body, producing images of organs, soft tissues, bone and virtually all other internal body structures.
When a person lies inside the magnetic tube, its magnetic field temporarily interacts with hydrogen nuclei in the water molecules of the human body.
The radio waves excite the spin of the nucleus of the hydrogen atoms, or protons, and the atoms produce very faint signals, which are used to create cross-sectional or three-dimensional images.
MRI is a benign, non-invasive treatment, as opposed to computerized tomography (CT) scans, whose high-energy X-rays ionize theatoms and can lead to cancer later in life.
MRIs are used to assess organs, tissue and bones; they can diagnose stroke and detect breast cancer, tumours, illnesses such as multiple sclerosis, and joint damage or disorders like arthritis.
They can be used to determine the size and function of the heart's chambers as well as any damage caused by heart attacks or diseases.
The hip joint is the most popular site for bone structure assessment, followed by the ankle, then the wrist.
Written by dee Hobsbawn-Smith for the Spring 2015 issue of the Green & White.
The man who once built telescopes as a youth and dreamed of working in aerospace is on the verge of sending a portable MRI to the International Space Station, bringing high-tech medical imaging in outer space one step closer to reality. "Our ultimate objective is to make magnetic resonance imaging (MRI) portable," said Sarty (Msc'90, PhD'95), professor and acting chair of the Division of Biomedical Engineering with the College of Arts and Science.
Sarty is still building things. A typical full-size MRI machine is a large, tubular magnet, about a metre-and-a-half long and 60 centimetres across—big enough to contain a human body. In the basement of the U of S Physics Building, Sarty and two graduate students are developing and fine-tuning a smaller version, large enough to scan a human wrist, with an internal diameter of 18 centimetres and 36 centimetres long. It weighs in at a relatively slender 50 kilograms, light enough to be considered for inclusion on the International Space Station, where volume, weight and electrical needs are strictly governed. The MRI Sarty is working on is small enough to be considered portable, and at $250,000, it costs a fraction of its larger cousin's $2 million price tag.
The project was one of five that won a 2014 Canadian Space Agency competition for new medical-study instruments. Two were selected for further funding in February, but the MRI project didn't make the cut. "It boils down to risk and dollars," he said wryly. "It would be awesome that Canada would be the first ever to put an MRI in space. Canada doesn't have a whole lot of firsts in space."
Sarty remains hopeful that he will receive additional funds toward production of the MRI in next year's allocation of the space agency's budget. It becomes a tight timeline, however. The space station's international collaboration among the European Space Agency, NASA, Japan and Russia is set to expire by 2025 at the latest, and Canada has not signed on to the agreement beyond 2020. Further, by 2030, the space station itself will be allowed to burn up in the Earth's atmosphere. "It will take a couple years to build the MRI," he said. "If we start building now, by 2017, it will get three years in operation."
Sarty is frequently asked, "Why build it, and what is its use?"
"The technological angle is that it's a challenge to get an MRI light enough to put on a rocket," he said in response. "That attracted me to the problem." A full-size MRI weighs about 11 tons. He explained that it is necessary to create a "very perfect" magnetic field, that requires many neodymium magnets. In the lab, he and his team are developing new technology to enable the MRI to work with imperfect magnets. "It will make the MRI lighter, which leads to greater portability.
"The other reason is that in space, astronauts lose muscle and bone mass because they don't use them," he added. "All the studies to understand why that happens are based on before and after measurement—MRIs before and after, but none while actually flying. It has been a guess to what happens in-between." Participating astronauts on the space station would be tested monthly by MRI if and when the machine reaches the stars.
Beyond space, a smaller, less expensive and portable MRI would be a worthwhile project on planet Earth. "What's really important is to increase accessibility of MRI and eliminate long waiting lines," Sarty said. The machine would not only make diagnostic imaging more affordable, it could be put to use in remote rural and northern communities, in developing countries, war zones and in disaster relief areas.
Sarty has retained his fondness for the sky and is a long-time active member of the Royal Astronomical Society of Canada. When he's not looking at stars, working in the lab or spending time with his family, he and Associate Professor Michael Bradley are busy co-supervising three fourth-year engineering students, Mitchell Tallon, Michael Dorma and Graham Scott, as they prepare their capstone project on measuring magnetic fields.
"My focus is along a businesslike direction," Sarty said. "Within two or three years, I hope to see this [portable MRI] on the market. This is the big project I'd like to see happen, either by working with manufacturers or by solving the technological problems ourselves.
How an MRI works
Magnetic resonance imaging (MRI) is a medical technique based on radio waves, magnets and computers, used to investigate the anatomy and physiology of the body, producing images of organs, soft tissues, bone and virtually all other internal body structures.
When a person lies inside the magnetic tube, its magnetic field temporarily interacts with hydrogen nuclei in the water molecules of the human body.
The radio waves excite the spin of the nucleus of the hydrogen atoms, or protons, and the atoms produce very faint signals, which are used to create cross-sectional or three-dimensional images.
MRI is a benign, non-invasive treatment, as opposed to computerized tomography (CT) scans, whose high-energy X-rays ionize theatoms and can lead to cancer later in life.
MRIs are used to assess organs, tissue and bones; they can diagnose stroke and detect breast cancer, tumours, illnesses such as multiple sclerosis, and joint damage or disorders like arthritis.
They can be used to determine the size and function of the heart's chambers as well as any damage caused by heart attacks or diseases.
The hip joint is the most popular site for bone structure assessment, followed by the ankle, then the wrist.
Written by dee Hobsbawn-Smith for the Spring 2015 issue of the Green & White.